ELECTRONOCATALYTIC INTENSIFICATION COMBUSTION OF GASEOUS FUEL.
DOI:
https://doi.org/10.15421/jchemtech.v31i1.271226Keywords:
electrocatalysi, burning; intensification; ga;, catalyst; dielectric barrier discharge.Abstract
Natural gas is a highly efficient energy carrier and a valuable chemical raw material. However, gas reserves are not unlimited. Therefore, there is a need to create technologies to reduce natural gas consumption and increase the productivity of its use. For this, it was proposed to use the directed action of an artificially created low-temperature plasma with an ordered movement of "slow" electrons in the presence of a heterogeneous catalyst and to determine the optimal conditions for these processes. The use of the electron-catalytic method in the processes of combustion allows spending a significantly smaller amount of energy on the process of initiating the primary endothermic stages due to the use of the energy of "slow" electrons, the formation of which is primarily influenced by the non-equilibrium plasma. The theoretical foundations of the process of electrocatalytic intensification combustion of gaseous fuel are considered, namely the influence of elastic and non-elastic collisions, ionization, temperature inhomogeneity between the gas flow and the low-temperature plasma flow, resonance of molecular vibration frequencies and electric discharge. The dependences of the temperature change of heated water on time at different voltages of the electric discharge, the coefficient of excess air and the composition of the catalyst during the electrocatalytic combustion of gaseous fuel are given.
References
Biletsky, V. S., Rozumny, P. V. (2015). World resources and reserves of natural gas. Collection of scientific works of FNGtP , 7 , 106–117.
Arutyunov, B.C., Krylov, O.V. (1998). [Oxidative transformations of methane]. Moscow, Russian Federation: Nauka (in Russian)
Itikawa, Y., Mason, N. (2005) Cross Sections for Electron Collisions with Water Molecules. J. Phys. Chem. Ref. Data, 34. (1), 1–22.
Hrabrov, V. A. (1983). [Physical and chemical processes in plasma chemical reactors]. Moscow, Russian Federation: Izd. MYTHS. (in Russian)
Avtaeva, S.V. (2009). [Barrier discharge. research and application]. Bishkek, Kyrgyzstan: Izd-vo KRSU (in Russian)
Kogelschatz, U. (2003). Dielectric-barrier Discharges: Their History, Discharge Physics, and Industrial Applications. Plasma Chemistry and Plasma Processing, 23(1), 1–46
Lim, M. T., Zulazlan, Shah Zulkifli, A. Z., Jayapalan, K. K., Chin, O. (2017). Development of a dimensionless parameter for characterization of dielectric barrier discharge devices with respect to geometrical features. Plasma Sci. Technol., 19(9), 095402
Syvin, S. (1971). [Oscillations of molecules and mean square amplitudes]. Moscow, Russian Federation: Mir (in Russian)
Hertzberg, H. (1974). [Spectra and structure of simple free radicals]. Moscow, Russian Federation: Mir (in Russian)
Rusanov, V. D., Friedman, A. A., Sholin, G. V. (1981) Physics of chemically active plasma with non-equilibrium oscillatory excitation of molecules. Successes of physical sciences, 134(2), 185–35 (in Russian)
Viazovyk, V. (2020). Electrocatalytic intensification of burning processes for hard and gaseous fuel. 3 International scientific and technical conference «Innovative development of resource-saving technologies and sustainable use of natural resources», Petrosani, Romania October, 26, 33–35.
Viazovyk, V. (2011). [Optimization of process of burning of gaseous fuel]. I International Scientific and Practical Conference "Advanced Development of Science and Technology", Przemysl, Polska. 2011, 97–99. (in Ukrainian)
Stolyarenko, H.S., Viazovyk, V.M., Vodyanyk, O.V. (2010). Electrocatalytic intensification of gaseous fuel combustion. Bulletin of the National Technical University, Kharkiv Polytechnic Institute, 13, 112–120 (in Russian)
Briegleb, G. (1964). Electron Affinity of Organic Molecules. Angewandte Chemie International Edition 3(9), 617–632
Amati, M., Stoia, S., Baerends, E. J. (2020) The Electron Affinity as the Highest Occupied Anion Orbital Energy with a Sufficiently Accurate Approximation of the Exact Kohn–Sham Potential. J. Chem. Theory Comput. 16(1), 443–452 https://doi.org/10.1021/acs.jctc.9b00981
Li, J, Duchemin, I, Roscioni, O. M., Friederich, P., Anderson, M., Como E. D., -Köhn, G. K., Wenzel, W., Zannoni, C., Beljonne, D., Blase, X., D'Avino, G. (2019). Host dependence of the electron affinity of molecular dopants. Mater. Horiz., 6, 107–114. https://doi.org/ 10.1039/c8mh00921j
He, J., Wen, X., Wu, L., Chen, H. (2022). Dielectric barrier discharge plasma for nanomaterials: Fabrication, modification and analytical applications. TrAC Trends in Analytical Chemistry, 156, November, 116715. https://doi.org/10.1016/j.trac.2022.116715
Li J., Ma, C., Zhu, S., Yu, F., Dai, B., Yang, D. (2019). A Review of Recent Advances of Dielectric Barrier Discharge Plasma in Catalysis. Nanomaterials, 9, 1428 https://doi.org/10.3390/nano9101428
Gorfinkiel, J., Morgan, L.A., Tennyson, J. (2002). Electron impact diccociative excitation of water within the adiabatic nuclei approximatiob. Journal of Physics B: Atomic, Molecular and Optical Physics, 35 543-555. https://doi.org/ 10.1088/0953-4075/35/3/309
Gorfinkiel, J.D. (2020). Electron collisions with molecules and molecular clusters. The European physical journal D, 7451. https://doi.org/10.1140/epjd/e2020-100550-7
Varghese, R. J., Kolekar, H.l, Kishore, V. R., Kumar, S. (2019). Measurement of laminar burning velocities of methane-air mixtures simultaneously at elevated pressures and elevated temperatures. Fuel, 257(1) 116120. https://doi.org/10.1016/j.fuel.2019.116120
Han, X., Wang, Z., Wang, S., Whiddon, R., He, Y., Lu, Yu, Konnov, A. A. (2019) Parametrization of the temperature dependence of laminar burning velocity for methane and ethane flames. Fuel, 239(1),1028–1037 https://doi.org/10.1016/j.fuel.2018.11.118
Han, X., Wang, Z., He, Y., Wang, S, Zhu, Y., Konnov, A.A. (2019). Over-rich combustion of CH4, C2H6, and C3H8 +air premixed flames investigated by the heat flux method and kinetic modelling. Combustion and Flame, 210, 339–349. https://doi.org/10.1016/j.combustflame.2019.09.009
Jia, Z., Yang, N., Sun, L., Zhao, Y., Li W., Luan, J, Lyu, F., Zhang, L.C, Kruzic, J.J., Kai, J.J, Huang, J.C., Lu J, Liu, C.T., Novel, А. (2020). Multinary Intermetallic as an Active Electrocatalyst for Hydrogen Evolution Advanced Materials, 2000385. https://doi.org/10.1002/adma.202000385
Bogaerts, A., Zhang, Q. Z., Zhang, Y. R., Laer, K. V., Wang, W. (2019). Burning questions of plasma catalysis: Answers by modelling. Catalysis Today, 337, 15, 3–14. https://doi.org/10.1016/j.cattod.2019.04.077
Uytdenhouwen, Y., Bal, K.M., Neyts, E.C., Meynen, V., Cool, P., Bogaerts, A. (2021) On the kinetics and equilibria of plasma-based dry reforming of methane. Chemical Engineering Journal, 405(1), 126630. https://doi.org/10.1016/j.cej.2020.126630
Uytdenhouwen, Y., Hereijgers, J., Breugelmans, T., Cool, P., Bogaerts, A. (2021) How gas flow design can influence the performance of a DBD plasma reactor for dry reforming of methane Chemical Engineering Journal, 405(1), February 126618 https://doi.org/.1016/j.cej.2020.126618
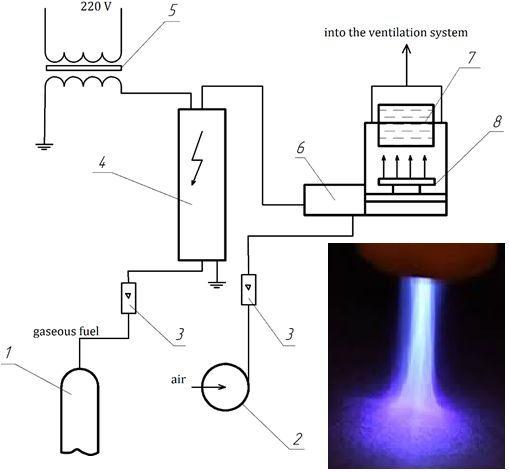
Downloads
Published
Issue
Section
License
Copyright (c) 2023 Oles Honchar Dnipro National University

This work is licensed under a Creative Commons Attribution 4.0 International License.
- Authors reserve the right of attribution for the submitted manuscript, while transferring to the Journal the right to publish the article under the Creative Commons Attribution License. This license allows free distribution of the published work under the condition of proper attribution of the original authors and the initial publication source (i.e. the Journal)
- Authors have the right to enter into separate agreements for additional non-exclusive distribution of the work in the form it was published in the Journal (such as publishing the article on the institutional website or as a part of a monograph), provided the original publication in this Journal is properly referenced
- The Journal allows and encourages online publication of the manuscripts (such as on personal web pages), even when such a manuscript is still under editorial consideration, since it allows for a productive scientific discussion and better citation dynamics (see The Effect of Open Access).