IMPROVED THERMODYNAMIC CYCLE OF A STEAM TURBINE PLANT
DOI:
https://doi.org/10.15421/jchemtech.v31i1.274768Keywords:
thermodynamic cycle, isobaric and isochoric steam superheating processes, steam turbine plant, plant modification, performance indicatorsAbstract
In modern steam turbine installations, steam is superheated in an isobaric process. As is known from technical thermodynamics, when the working fluid is heated from a given state to the same temperature, the heat in the isobaric process is greater than the heat of the isochoric one. In addition, the expansion of the working fluid during the isobaric process of heat supply is harmful, since in this case no external work is performed, that is, the main property of the working fluid is lost – its physical expansion in order to obtain external mechanical work. In an isochoric process, steam, like a mechanical spring, accumulates more mechanical energy at a lower cost of heat. Therefore, it is proposed in steam turbine plants that both primary superheating and subsequent steam superheating processes be carried out isochorically. Comparative calculations of the characteristics of steam turbine installation, operating according to the Rankine cycle with primary superheated steam up to a pressure of 6.0 MPa and a temperature of 600 °C, at a steam pressure in the condenser of 0.004 MPa, with the characteristics of an installation with an isochoric steam superheating process with the same initial data, showed significant energy and economic advantages of the latter in comparison with basic. So, with a lower fuel consumption in the modified installation (by 10.1 %), its specific work increased by 3.9 %, and thermal efficiency – by 11.2 %. Even more significant energy and economic advantages have a modified STP with one additional reheat of steam: with a lower fuel consumption by 15.9 %, the specific work increased by 6.8 %, and the thermal efficiency increased by 18.8 %. Due to the smaller volumes of steam at the end of the expansion, the weight and size parameters of the turbines and the condenser of the modified unit are reduced by 5.2 % compared to the base unit. Considering the above conclusions and the scale of use of STP at modern power plants, where up to ten intermediate steam reheats are used, the proposed modernization of their thermodynamic cycles guarantees even greater energy and economic effects.
References
Kirillin V.A., Sychev, V.V., Sheindlin, A.E. (2016). [Technical thermodynamics]. Publishing House MEI. (In Russian).
Lemmon, E.W, Huber, M.L, McLinden, M.O. (2007). NIST Reference Fluid Thermodynamic and Transport Properties. REFPROP, Version 8.0. Gaithersburg.
Vasserman, O.A., Slinko, O.G. (2019). [Technical thermodynamics and heat transfer]. Odessa: Phoenix. (In Russian).
Vasserman, O.A., Slinko, O.G., Shutenko, M.A. (2020). [Innovative thermodynamic cycles of power plants]. Odessa: Phoenix (In Russian).
Vasserman, O.A., Slynko, O.G. (2018). [Improving the efficiency of steam turbine installations]. Tekhnicheskie Gazy – Industrial gases, 1, 39–42. (In Russian).
Laux, Ch., Gotter, A., Eckert, F., Neef, M. (2022). Experimental results of a low-pressure steam Rankine cycle with a novel water lubricated radial inflow turbine for the waste heat utilization of internal combustion engines. Energy Conversion and Management, 271, 116265. https://doi.org/10.1016/j.enconman.2022.116265
Elahi, A. E., Mahmud, T., Alam, M., Hossain, J., Biswas, B. N. (2022). Exergy analysis of organic Rankine cycle for waste heat recovery using low GWP refrigerants. International Journal of Thermofluids, 16, 100243 https://doi.org/10.1016/j.ijft.2022.100243
Kasaeian, A., Shamaeizadeh, A., Jamjoo, B. (2022). Combinations of Rankine with ejector refrigeration cycles: Recent progresses and outlook. Applied Thermal Engineering, 211, 118382 https://doi.org/10.1016/j.applthermaleng.2022.118382
Köse, Ö., Koç, Y., Yağli, H. (2022). Is Kalina cycle or organic Rankine cycle for industrial waste heat recovery applications? A detailed performance, economic and environment based comprehensive analysis. Process Safety and Environmental Protection, 103, 421–437. https://doi.org/10.1016/j.psep.2022.05.041
Reshaeel, M., Javed, A., Jamil, A., Ali, M., Mahmood, M., Waqas, A. (2022). Multiparametric optimization of a reheated organic Rankine cycle for waste heat recovery based repowering of a degraded combined cycle gas turbine power plant. Energy Conversion and Management, 254, 115237.https://doi.org/10.1016/j.enconman.2022.115237
Kalan, A. Sh., Heidarabadi, Sh., Khaleghi, M., Ghiasirad, H. (2023). Biomass-to-energy integrated trigeneration system using supercritical CO2 and modified Kalina cycles. Energy and exergy analysis. Energy, 270, 126845. https://doi.org/10.1016/j.energy.2023.126845
Akimoto, R., Yamaki, T., Nakaiwa, M., Matsuda K. (2021). Evaluation of a power generation system that integrates multiple Kalina cycles and absorption heat pumps. Case Studies in Thermal Engineering, 20, 101363. https://doi.org/10.1016/j.csite.2021.101363
Devi Parvathy, S., Varghese, J. (2021). Energy analysis of a Kalina cycle with double turbine and reheating. Materials Today: Proceedings, 47(15), 5045–5051. https://doi.org/10.1016/j.matpr.2021.04.636
Roeinfard, N., Moosavi, A. (2022). Thermodynamic analysis and optimization of the organic Rankine and high-temperature Kalina cycles for recovering the waste heat of a bi-fuel engine. Fuel, 322, 124174. https://doi.org/10.1016/j.fuel.2022.124174
Zhang, Sh., Hao, X., Chen, Ya., Wu, J. (2021). Thermodynamic study on power and refrigeration cogeneration Kalina cycle with adjustable refrigeration temperature. International Journal of Refrigeration, 131, 706-713 https://doi.org/10.1016/j.ijrefrig.2021.05.014
Shifan Yang, Fang Fang, Yaping Chen, Jiafeng Wu, Shaobo Zhang (2021). Study on approaches of extending refrigeration capacity range of parallel power/refrigeration cogeneration Kalina cycle system. International Journal of Refrigeration, 131, 426–436. https://doi.org/10.1016/j.ijrefrig.2021.06.003
Mahmoudi, S.M.S., Pourreza, A., A.D. Akbari, M. Yari (2016). Exergoeconomic evaluation and optimization of a novel combined augmented Kalina cycle/gas turbine-modular helium reactor. Applied Thermal Engineering, 109(A), 109–120. https://doi.org/10.1016/j.applthermaleng.2016.08.011
Lavrenchenko, G.K., Vasserman, O.A. (2018). [New generation of geothermal stations working on Kalina cycle using ammonia-water solution]. Tekhnicheskie Gazy – Industrial gases, 2, 3–12. (In Russian).
Vasserman, O.A., Slyn'ko, A.G. (2018). [Rise economy of steam turbine plants]. Tekhnicheskie Gazy – Industrial gases, 1, 49–52. (In Russian).
Vasserman, O.A., Slyn'ko, A.G. (2018). [Increasing the efficiency and power of internal combustion engines. 1. Internal combustion engine cycle with the release of exhaust gases into the atmosphere]. Tekhnicheskie Gazy – Industrial gases, 3, 34–37. (In Russian).
Vasserman, O.A., Slyn'ko, A.G. (2018). [Increasing the efficiency and power of internal combustion engines. 2. Cycle with air cooling, which is supplied to the engine cylinders]. Tekhnicheskie Gazy. – Industrial gases, 4, 43–47. (In Russian).
Vasserman, O.A., Slyn'ko, A.G. (2019). [Increasing the efficiency and power of internal combustion engines. 3. Cycle with gas turbine supercharging and cooling of the air supplied to the internal combustion engine]. Tekhnicheskie Gazy – Industrial gases, 1, 53–57. (In Russian).
Vasserman, O.A., Slyn'ko, A.G. (2019). [Increasing the efficiency and power of internal combustion engines. 4. Cycle with gas turbine supercharging, cooling of the air supplied to the internal combustion engine, and expansion of gases in the turbine to a pressure below atmospheric]. Tekhnicheskie Gazy. – Industrial gases, 2, 44–48. (In Russian).
Robert M. Garceau, William J. Wepfer (1983)/ Thermoeconomic Optimization of a Rankine Cycle Cogeneration System. ACS Symposium Series, 235(13), 263–288. https://doi.org/10.1021/bk-1983-0235.ch013
Davood, A., Afshin, M. A., Gholamreza S., Masoud T. A. (2022). Analysis of Different Organic Rankine and Kalina Cycles for Waste Heat Recovery in the Iron and Steel Industry, ACS Omega, 7, 50. https://doi.org/10.1021/acsomega.2c03922
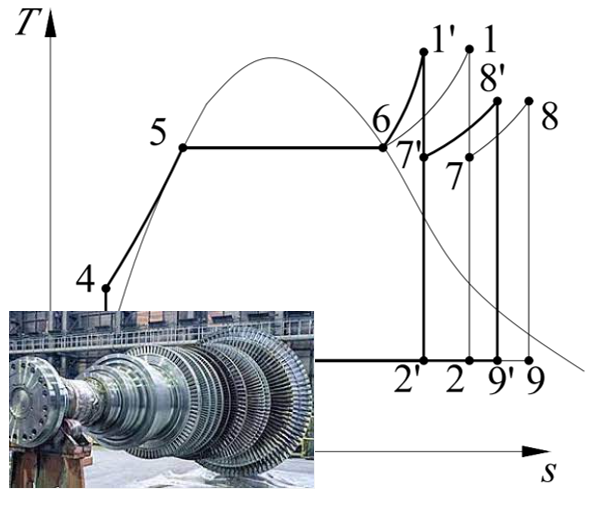
Downloads
Published
Issue
Section
License
Copyright (c) 2023 Oles Honchar Dnipro National University

This work is licensed under a Creative Commons Attribution 4.0 International License.
- Authors reserve the right of attribution for the submitted manuscript, while transferring to the Journal the right to publish the article under the Creative Commons Attribution License. This license allows free distribution of the published work under the condition of proper attribution of the original authors and the initial publication source (i.e. the Journal)
- Authors have the right to enter into separate agreements for additional non-exclusive distribution of the work in the form it was published in the Journal (such as publishing the article on the institutional website or as a part of a monograph), provided the original publication in this Journal is properly referenced
- The Journal allows and encourages online publication of the manuscripts (such as on personal web pages), even when such a manuscript is still under editorial consideration, since it allows for a productive scientific discussion and better citation dynamics (see The Effect of Open Access).