DETERMINATION OF CONCRETE THERMO-CHEMICAL DESTRUCTION REGULATIONS UNDER THE INFLUENCE OF HIGH TEMPERATURES
DOI:
https://doi.org/10.15421/jchemtech.v32i2.298636Keywords:
thermochemical cracking; destruction of concrete; change in the chemical composition of concrete.Abstract
The study solved the problem of investigating the mechanism of concrete destruction under the influence of high temperatures as a result of an explosion. The destruction of the concrete floor after the impact of the projectile was established, which is explained by the effect of high temperatures. Concrete samples at different distances from the epicenter had a melted surface and a change in color. The use of the TPD-MS method showed that CO was released from a sample located 1.5 m from the epicenter with an intensity of 0.016 at the temperature of 100 °С; from a distance of up to 2 m with an intensity of 0.006; from the control sample with an intensity of 0.01 at 583 °С. A control sample of concrete when heated to 583 °С emitted CO2 with an intensity of 0.16; the sample was obtained at a distance of up to 2 m with an intensity of 0.1 at 537 °С. In the concrete samples obtained at a distance of 0.5 m to 2 m, CO2 emission was minimal with an intensity of 0.04 at 537 °С. The carbon content (СО, СО2) in concrete decreases when approaching the epicenter of the explosion. The highest intensity of water evaporation of 0.8 when heated to 100 °С was observed from a concrete sample within a radius of up to 1.5 m from the epicenter. Sulfur was released in small amounts when heated to 1000 °С in all concrete samples. SO2 and SO compounds were isolated from a sample obtained at a distance of 1.5 from the explosion. The feature of the experiment is the investigation of thermomechanical and thermochemical changes in concrete under the influence of high temperatures. The conducted research is distinguished by the use of scanning electron microscopy and TPD-MS methods for the study of structural and chemical changes in concrete. The results of the experiment can be used to determine the degree of destruction of concrete after explosion and fire for the restoration of the building.
References
Pozdjejev, S. V., Otrosh, Ju. A., Omeljchenko, A. M., & Shhipecj, S. D. (2014). [A method for assessing the fire resistance limit of reinforced concrete beams by interpreting the results of their fire tests]. Zbirnyk naukovykh pracj Donbasjkogho derzhavnogho tekhnichnogho universytetu, (1), 119–127.
Ghyvljud, M. M. Parkhomenko V.-P. O., Brajchenko S. P. (2016). [Temperature dependence of strength properties of concrete based on composite cement]. Vydavnyctvo Ljvivsjka politekhnika. 844, 47–52
Alhamad, A., Yehia, S., Lublóy, É., Elchalakani, M. (2022). Performance of Different Concrete Types Exposed to Elevated Temperatures: A Review. Materials, 15(14), 5032. https://doi.org/10.3390/ma15145032
Mohammed, H., Ahmed, H., Kurda, R., Alyousef, R., Deifalla, A. F. (2022). Heat-Induced Spalling of Concrete: A Review of the Influencing Factors and Their Importance to the Phenomenon. Materials, 15(5), 1693. https://doi.org/10.3390/ma15051693
Wyrzykowski, M., Terrasi, G., Lura, P. (2020). Chemical prestressing of high-performance concrete reinforced with CFRP tendons. Composite Structures, 239, 112031https://doi.org/10.1016/j.compstruct.2020.112031
Wyrzykowski, M., Terrasi, G., Lura, P. (2018). Expansive high-performance concrete for chemical-prestress applications. Cement and Concrete Research, 107, 275–283.https://doi.org/10.1016/j.cemconres.2018.02.018
Chaojie Song, C., Zhang, G., Lu, Z., Li, X., Zhao, X. (2023) Fire resistance tests on polypropylene-fiber-reinforced prestressed concrete box bridge girders. Engineering Structures, 115800, https://doi.org/10.1016/j.engstruct.2023.115800
Liu, J. C., Tan, K. H., Yao, Y. (2018). A new perspective on nature of fire-induced spalling in concrete. Construction and Building Materials, 184, 581–590, https://doi.org/10.1016/j.conbuildmat.2018.06.204.
Miah, M. J., Kallel, H., Carré, H., Pimienta, P., La Borderie, C. (2019). The effect of compressive loading on the residual gas permeability of concrete. Construction and Building Materials, 217, 12–19. https://doi.org/10.1016/j.conbuildmat.2019.05.057
Du, H., Zhang, M. (2020). Experimental investigation of thermal pore pressure in reinforced C80 high performance concrete slabs at elevated temperatures. Construction and Building Materials, 260, 120451, https://doi.org/10.1016/j.conbuildmat.2020.120451
Choe, G., Kim, G., Yoon, M., Hwang, E., Nam, J., Guncunski, N. (2019). Effect of moisture migration and water vapor pressure build-up with the heating rate on concrete spalling type. Cement and Concrete Research, 116, 1–10. https://doi.org/10.1016/j.cemconres.2018.10.021.
Li, Y., Pimienta, P., Pinoteau, N., Tan, K. H. (2019). Effect of aggregate size and inclusion of polypropylene and steel fibers on explosive spalling and pore pressure in ultra-high-performance concrete (UHPC) at elevated temperature. Cement and Concrete Composites, 99, 62–71. https://doi.org/10.1016/j.cemconcomp.2019.02.016
Bilir, T., Kockal, N. U., Khatib, J. M. (2020). Properties of SCC at elevated temperature. In Self-Compacting Concrete: Materials, Properties and Applications, Woodhead Publishing https://doi.org/10.1016/B978-0-12-817369-5.00008-8.
Xu, L., Wang, J., Li, K., Lin, S., Li, M., Hao, T., Ling, Z., Xiang, D., Wang, T. (2022). A systematic review of factors affecting properties of thermal-activated recycled cement. Resources, Conservation and Recycling, 185, 106432. https://doi.org/10.1016/j.resconrec.2022.106432.
Maier, M., Saxer, A., Bergmeister, K., Lackner, R. (2020). An experimental fire-spalling assessment procedure for concrete mixtures. Construction and Building Materials, 232, 117172. https://doi.org/10.1016/j.conbuildmat.2019.117172.
Murphy, C. J., Ardy Nugroho, F. A., Härelind, H., Hellberg, L., Langhammer, C. (2021). Plasmonic Temperature-Programmed Desorption. Nano letters, 21(1), 353–359. https://doi.org/10.1021/acs.nanolett.0c03733.
Bozhokin, M. S., Bozhkova, S. A., Rubel, A. A., Sopova, J. V., Nashchekina, Y. A., Bildyug, N. B., & Khotin, M. G. (2021). Specificities of Scanning Electron Microscopy and Histological Methods in Assessing Cell-Engineered Construct Effectiveness for the Recovery of Hyaline Cartilage. Methods and protocols, 4(4), 77. https://doi.org/10.3390/mps4040077
Sundin, M., Hedlund, H., Cwirzen, A. (2023). Eco-Concrete in High Temperatures. Materials, 16(12), 4212. https://doi.org/10.3390/ma16124212
Sodol, K. A., Kaczmarek, Ł., Szer, J. (2020). Fire-Temperature Influence on Portland and Calcium Sulfoaluminate Blend Composites. Materials, 13(22), 5230. https://doi.org/10.3390/ma13225230
Wróblewska, J., Kowalski, R. (2020). Assessing concrete strength in fire-damaged structures. Construction and Building Materials, 254, 119122. https://doi.org/10.1016/j.conbuildmat.2020.119122.
Shumuye, E. D., Zhao, J., Wang, Z. (2021). Effect of the curing condition and high-temperature exposure on ground-granulated blast-furnace slag cement concrete. International Journal of Concrete Structures and Materials, 15, 1-20. https://doi.org/10.1186/s40069-020-00437-6.
Reddy, V. G. P., Tadepalli, T., Pancharathi, R. K. (2022). Surface imaging based non-destructive assessment of concrete deterioration using hue-saturation-intensity colour space. Measurement, 197, 111311. https://doi.org/10.1016/j.measurement.2022.111311.
Shkromada, O., Ivchenko, V., Chivanov, V., Tsyhanenko, L., Tsyhanenko, H., Moskalenko, V., Kyrchata, І., Shersheniuk, O., Litsman, Y. (2021). Defining patterns in the influence exerted by the interelated biochemical corrosion on concrete building structures under the conditions of a chemical enterprise. Eastern-European Journal of Enterprise Technologies, 2(6(110), 52–60. https://doi.org/10.15587/1729-4061.2021.226587.
Shkromada, O., Fotina, T., Petrov, R., Nagorna, L., Bordun, O., Barun, M., Babenko, O., Karpulenko, M., Tsarenko, T., Solomon, V. (2021). Development of a method of protection of concrete floors of animal buildings from corrosion at the expense of using dry disinfectants. Eastern-European Journal of Enterprise Technologies, 4(6(112), 33–40. https://doi.org/10.15587/1729-4061.2021.236977
Raghav, M., Park, T., Yang, H. M., Lee, S. Y., Karthick, S., Lee, H. S. (2021). Review of the Effects of Supplementary Cementitious Materials and Chemical Additives on the Physical, Mechanical and Durability Properties of Hydraulic Concrete. Materials, 14(23), 7270. https://doi.org/10.3390/ma14237270.
Shkromada, O., Ivchenko, V., Chivanov, V., Shvets, O., Moskalenko, V., Kochenko, A., Babenko, O., Kharchenko, Y., Pikhtirova, A., Yurchenko, O. (2022). Determining changes in the mineral composition of concrete due to chemical corrosion in a sulfate environment. Eastern-European Journal of Enterprise Technologies, 6(6(120), 42–50. https://doi.org/10.15587/1729-4061.2022.268627.
Pasztetnik, M., Wróblewski, R. (2021). A Literature Review of Concrete Ability to Sustain Strength after Fire Exposure Based on the Heat Accumulation Factor. Materials, 14(16), 4719. https://doi.org/10.3390/ma14164719
Chai, L., Chen, B., Guo, L., Ren, B., Chen, Z., Huang, T. (2023). Effect of Ambient Temperature on the Mechanical Properties of High Ductility Concrete. Materials, 16(6), 2465. https://doi.org/10.3390/ma16062465
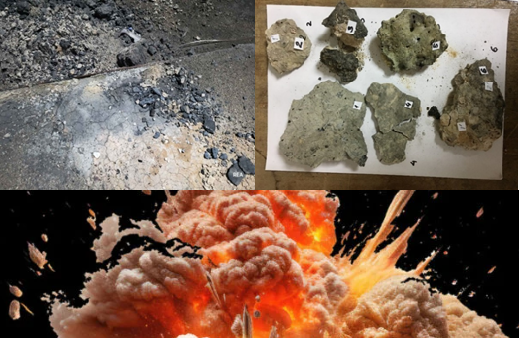
Downloads
Published
Issue
Section
License
Copyright (c) 2024 Oles Honchar Dnipro National University

This work is licensed under a Creative Commons Attribution 4.0 International License.
- Authors reserve the right of attribution for the submitted manuscript, while transferring to the Journal the right to publish the article under the Creative Commons Attribution License. This license allows free distribution of the published work under the condition of proper attribution of the original authors and the initial publication source (i.e. the Journal)
- Authors have the right to enter into separate agreements for additional non-exclusive distribution of the work in the form it was published in the Journal (such as publishing the article on the institutional website or as a part of a monograph), provided the original publication in this Journal is properly referenced
- The Journal allows and encourages online publication of the manuscripts (such as on personal web pages), even when such a manuscript is still under editorial consideration, since it allows for a productive scientific discussion and better citation dynamics (see The Effect of Open Access).